IV. Biophysics
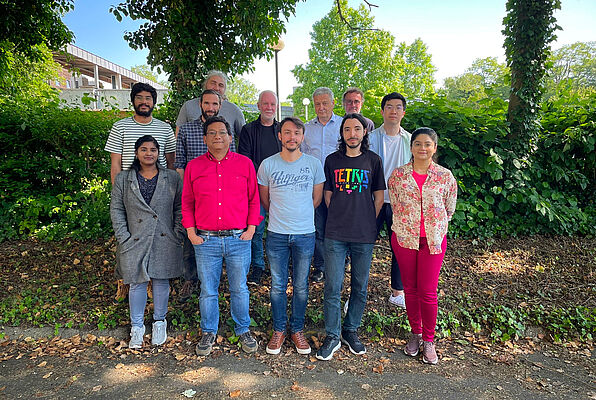
Biophysics
Researchers and Engineers: Francois ANQUEZ, Emmanuel COURTADE, Marc LEFRANC, Laurent HELIOT, Jean PESEZ, Benjamin PFEUTY, Franck RIQUET, Raoul TORERO, Bernard VANDENBUNDER
PhDs and postdocs: Noémie GOUSPILLOU, Abdelkrim HANNOU, Eden HASEEB, Alexandre LEBEL, Henri TRUONG, Alan VANDENBERGHE,
One distinguishing aspect of the physical biology research conducted at PhLAM lies in the integration of imaging, biophysics, and modeling approaches to investigate the functional dynamics of signaling networks. Leveraging the comprehensive expertise of DYSCO in optics and nonlinear dynamics spanning several decades, our research capitalizes on these interdisciplinary techniques to investigate a wide range of cellular functions, encompassing transcription regulation, stress response, stem cell differentiation, programmed cell death, or the circadian clock. Despite the diversity and differences of these cellular functions, common questions are addressed regarding the molecular orchestration of cellular processes. A significant part of research is dedicated to instrumental and methodological development ranging from optofluidics, biosensing and spatiotemporal microscopy techniques to a wide spectrum of modeling techniques.
Cellular response to stress is a typical and ubiquitous process that involves signal processing, adaptation dynamics, and phenotypic heterogeneity. This activity emerged from the ability to induce acute thermal and oxidative stress through laser. In response to thermal stress, live imaging measurement of transcriptional response has allowed extensive characterisation of the adaptation dynamics at both single-cell and population levels. Data and model analysis explain the observed phenotypic heterogeneity by the interplay of an ultrasensitivity mechanism and variations in the expression levels of chaperones controlled by the HSF1 factor (Guilbert et al., Febs J, 2020; Guilbert et al., Int J Mol Sci, 2023). Coupling the proposed model of heat shock response with phenomenological models of cell death has enabled to capture various striking features of survival response curves to diverse dose profile of temperature (Ladjimi et al., Int J Hyperth, 2019, Labavic et al., Int J Hyperth, 2020,Pfeuty et al., Phys Biol, 2021). These findings have motivated the development of generic cell stress-response models combining properties of adaptation, fate decision and noise, to show how general features of dose-response curves tightly depend on system timescales and nonlinearities (Labavic et al., J. Theor Biol, 2019,Hurbain et al., Sci Rep, 2020).
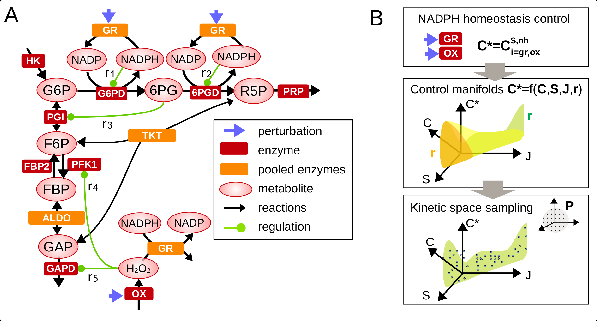
A keen interest has been also developed regarding the cellular response to a metabolic stress induced by reactive oxygen species (Simiuc et al., Biorxiv, 2020). The response to oxidative stress involves indeed coordinated regulation of metabolic pathways to generate NADPH antioxidant species. Original stochastic inference methods were first developed to estimate metabolic fluxes from isotopic labeling data (Thommen et al., Metab Eng, 2022). Development of kinetic models of metabolic networks revealed some regulatory principles based on cooperative feedback regulation dedicated to optimally responding to widely different stress levels (Hurbain et al., Iscience, 2022; Pfeuty et al., Metabolites, 2023).
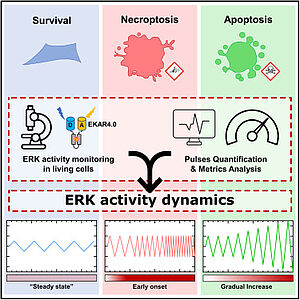
With the integration of Franck Riquet and in the context of a collaboration with Ghent University, a new research activity explores the role of master kinase regulators (MAPK/ERK) in various cell-fate decision processes, notably in programmed cell death such as apoptosis, necroptosis and pyroptosis. This activity builds upon several original paradigms. For instance, fate progression and determination between different types of cell death upon TNF signals can be determined by the activity pattern of MAPK (Sipieter et al., Iscience, 2021). Also, pore formation during cell death, such as secondary necrosis, is proposed to be a highly regulated process (De Schutter et al., Cell Mol Life Sci, 2022) and extracellular vesicles are prone to play a key role for cell-to-cell communication during cell death (Cappe et al., J Extracell Vesicles, 2023). These projects heavily rely on live-cell fluorescence imaging (Ladjik et al., Front Cell Death, 2023, Vecchia, acs sens, 2021).
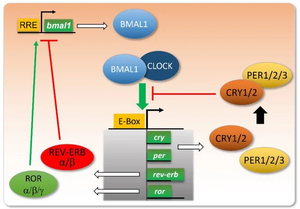
In 2019-2023, we worked on providing experimental evidence of a theoretical prediction that we made previously (Woller et al., Cell Reports 2016). Our numerical simulations suggested that the AMPK metabolic sensor is a key input to the mammalian circadian clock, that altered AMPK signaling is responsible for liver clock disruption such as observed upon a high-fat diet or in some metabolic disorders, and that PGC1a is a key mediator in the associated pathway. We have thus set up experiments to test our hypothesis using U2OS cells, a well established cell line for circadian studies. We finally established that activating AMPK in U2OS cells strongly enhances the amplitude of clock oscillations, significantly lengthens the clock period and advances or delays the clock phase depending on the administration time, all properties that are the signature of an important clock synchronizer. We also designed a minimal mathematical model reproducing surprisingly well clock oscillations in mouse livers. Through a systematic screen of all input parameters, we showed that the dramatic change in clock oscillations observed when subjecting mice to a high-fat most probably results from an elevated transcription of the key clock activator Bmal1, totally consistent with our experimental findings (Vandenberghe et al., Pharmaceutics 2022). These results obtained during the reference period have led to three manuscripts that are currently under redaction.
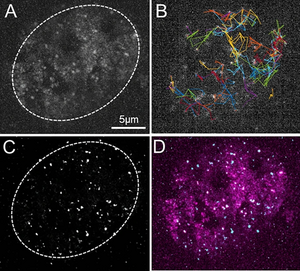
Understanding the molecular mechanisms underlying the main functions of living organisms and their regulation is a major challenge in biology. Our functional imaging approach consists of measuring activities, forces and kinetics at the molecular level in living cells, quantifying them and modeling the results using the laws of physics and chemistry. Transcription regulation is a central mechanism in the dynamics of gene networks. We have developed tools to understand the mechanics of spatiotemporal regulation of gene transcription by RNA polymerase II (RNAP II), focusing on the release of the transcriptional pause where transcription is started, then paused until a specific signal is received. Interestingly, actors of transcription pause release are often impaired in their function in several pathologies (Furlan et al., Med&Sci, 2018). Transcriptional pausing is a key step for different regulatory networks studies in the biophysical group, including: cell differentiation and destiny, stress response, and circadian clock involvement.
Our approaches are based on quantitative microscopy methods: fluorescence fluctuation measurement as FCS (Fluorescence Correlation Spectroscopy) and ICS (image Correlation Spectroscopy) ; super resolution technique for Single Particle Tracking; interactions between molecules in living cells by FRET-FLIM. For analyzing molecular interactions and characterizing the size of interaction regions, we have developed a new method (FICS) based on FRET-FLIM and ICS (Bidaux et al, Biophys. J, 2018 supported by a Nikon contract). Classically, the mobility of molecules in cells encompasses several modes of diffusion. The recovery of quantitative data on the rapid and slow diffusion of molecules is essential to understand the dynamics of molecular events on large scales (between 0.1 and 15 µm2/s for proteins in the nucleus). In order to overcome the limitations of each individual technique and observe molecular mobility on a large diffusion scale, we have developed a method based on the exploitation of SPT and FCS measurements (Fournier et al., Sc.Rep., 2023). We have also continued to develop tools and methods for analyzing FLIM and FCS/SPT data. We have worked with A. Leray (Inst. Carnot Dijon) to develop a new way for FLIM analysis (Net-Phasor) using deep learning algorithm (CNN) (Héliot & Leray, Sc. Rep. 2021, software licence: IDDN.FR.001.140001.000.S.P.2021.000.21000, 2021). Over the last 5 years, we have developed hundreds of plasmids and more than twenty cell lines expressing proteins associated with genetically encoded fluorescent markers and functional mutations. We also set up labelling strategies based on CRISP for locating specific genes (Cas9 strategy) and their transcription (Cas13 strategy) in living cells.
We took advantage of the combination of these different methods to study the recruitment mechanism of RNA polymerase II to target genes and decipher the regulatory mechanism based on the release of pauses in CPER Photonics4Society project (Bidaux et al, Biophys. J, 2018 ; Furlan et al., Biophysic. J, 2019). Through a cross-analysis of FCS and SPT, we were able to highlight four different motility states of RNAP II including two subdiffusion modes and revealed RNAP II oversampling of nuclear subdomains. Based on these data we propose a new model of spatio-temporal organization of transcription regulation (Fournier et al., Sc.Rep., 2023 ; ANR ABC4M). Taking the advantage of our microscopy techniques, we have collaborated with A. Portier’s team, UMR 8161, to determine whether transcriptional co-factor Ets-1 overexpression in cancer cells promotes angiogenesis by creating a pro-genetic environment for endothelial cells. Ets-1 appeared as a key regulator of the angiogenic potential of breast cancer cells, promoting their ability to induce the morphogenesis of endothelial cells and also to physically interact with them. Ets-1 modulation in breast cancer cells qualitatively modified the angiogenic profile of experimental tumors in vivo. (Furlan et al., Int J. Enco., 2019). In collaboration with laboratories UGSF in ULille and DIMES in UBologna, we used our microscopy technique to study the glycosylation by B4GALNT2 involved in colon cancer and genetic diseases (Groux-Degroote et al., FEBS 2018; ANR GalFISH) and the dynamics of a calcium channel family (TRPM8) which is involved in the interactions between the Endoplasmic Reticulum and the mitochondria, involved in various cancers including prostate (Bidaux et al., BBA Mol. Cell. Biol., 2018). Additionally, we have contributed to show that the pro-apoptotic fragment generated by the cleavage of the MET caspase is localized in the membrane region associated with mitochondria. This fragment triggers a transfer of calcium from the endoplasmic reticulum to the mitochondria, which is decisive for the apoptotic action of the receptor (Duplaquet et al., Elife, 2020).
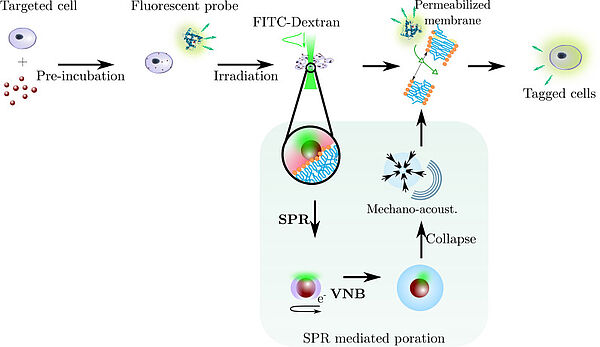
In the context of the ERC project 'Nanobubbles' led by Ghent University, the research group has developed a new optofluidic method that allows bringing gold nanoparticles close to cells for photoporation while avoiding direct contact with the cells through hydrodynamic optimization in a multiple-flow device. This technique has successfully transfecting cells with an efficiency of approximately 70% without a significant reduction in cell viability at a flow rate ranging from 103 to 104 cells per minute (Layachi et al, J. Nanobiotech, 2023). This device, aimed at producing therapeutic cells, has been patented and licensed to the spin-off Trince in Ghent (K. Braeckmans, WO2020221883A1, 2020). Furthermore, the expertise developed in this project has led to an interdisciplinary study with Franco-British chemists to develop a new catalytic system based on gold nanoparticles which, under the influence of light, heat their environment, thereby making the oxidation of hydrocarbons, a common but energy-intensive industrial reaction, gentler (Feng et al, Green Chemistry, 2023 and news from the CNRS Institute of Chemistry).
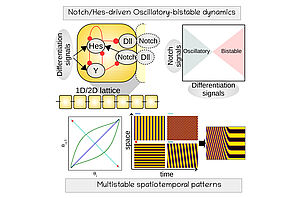
Regulatory models of pluripotent and mutlipotent stem cell differentiation have revealed how intercellular signaling patterns are readout by intracellular dynamics to generate developmental trajectories in space and time
(Refs: Pfeuty, Development, 2015; Pfeuty and Kaneko, Phys Biol, 2016, Pfeuty et al., Biophys J, 2018; Pfeuty, J Theor. Biol, 2022).
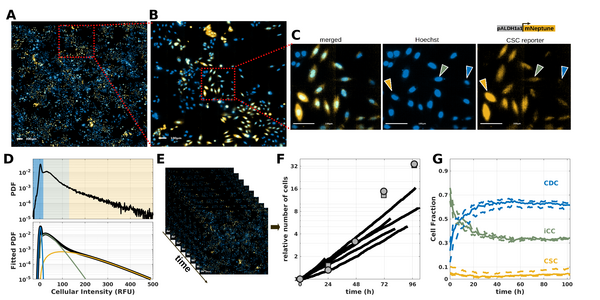
Alongside these modeling efforts, a collaboration has been in place since 2019 with Canther laboratory and supported by Hauts-de-France Funding. This collaboration has begun with the implementation of imaging tools based on the use of the fluorescent reporter ALDH (Bidan et al., Proteomics, 2019) and bioinformatic tools with the development of R tool to analyze CpG methylation (Denoulet et al., Bioinformatics 2023), and aims to understand the spatial self-organization of cancer stem cell niches (Brulé et al, Biorxiv, 2024).