2) Exotic doping
a) Bismuth-doped fibres
Since the end of 2005, the team uses MCVD to fabricate aluminosilicate, germanosilicate and phosphosilicates preforms doped with bismuth. The relatively recent interest in this doping (the first report of an IR emission in this kind of glass dates back to 2001) lies in the possibility of obtaining a laser effect in the range 1.1-1.5 µm, depending on the host-matrix composition. Beyond the fact that this spectral range is not accessible to rare-earth ions in silica, the interest in this dopant lies in its light emission bands that are large of several hundreds nanometers. Moreover, the shape of this emission is strongly dependent on the excitation wavelength. It quickly appeared possible to exploit this luminescence in order to achieve fibred lasers. Hence, we were the first ones in 2007 to demonstrate a laser efficiency higher than 20 % in this type of doped fibre. Since then, the work has been pursued, in particular by the study of the optical properties of bismuth when introduced in a pure sol-gel-derived silica matrix and by sudying the impact of the atmosphere on emission/absorption properties of MCVD preforms. This subject is founded since 2013 by ANR (project BOATS).
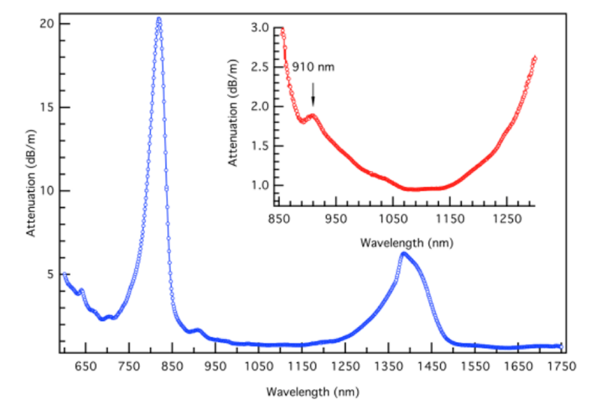
Attenuation curve of air/silica fibre with bismuth-doped core. Two main absorption bands are evidenced around 800 and 1400 nm.
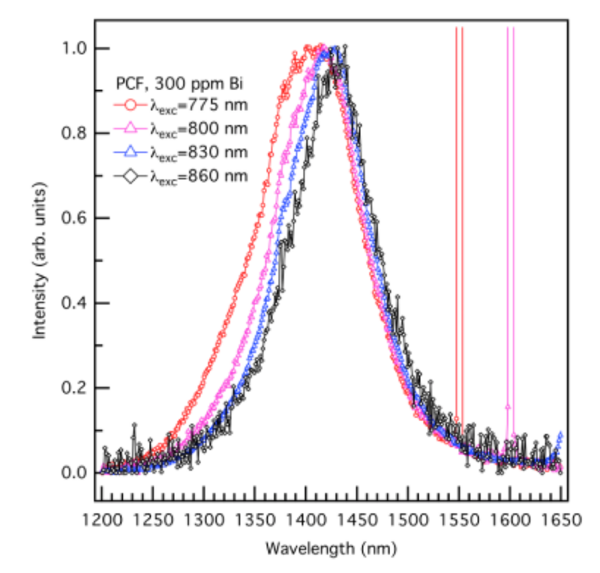
Photoluminescence spectra recorded in fibres for different excitation wavelengths. Emission of bismuth centers appears strongly red-shifted as compared to what can be observed in aluminosilicate fibres.
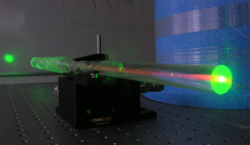
A bismuth-doped optical preform.
b) Doping fibres with metal nanoparticles
It is well-known that noble metal ou semiconductor nanoparticles (NPs) exhibit high nonlinear optical coefficients, especially in the neighbourhood of their resonance (surface plasmon resonance for metals and exciton for semiconductors). NPs may also be studied in the scope of energy transfer towards active ions in order to enhance amplifier’s or laser’s efficiency. However, these objects, widely studied in thin films or in bulk materials, had never been introduced in the core of an optical fiber in a clearly demonstrated manner. This quasi-absence of realisation is partly ascribable to the difficulty of preserving nanoparticles during the different steps of glass fabrication and fibre drawing . In effect, while melting temperature of metallic gold is close to 1064°C, the glass-working temperature is around 2000°C. In order to attempt to stabilise gold nanoparticles in the core of a fibre, a first stategy is to take advantage of the sol-gel synthesis of silica glass, which limits the impact of high temperature treatments. This approach, developped in Lille since 2002 in the case of monoliths, consists of a chemical way yielding nanoporous glasses that may be impregnated by solutions contening the desired precursors. The use of a gold precursor or of a solution contening gold nanoparticles actually leads to drawable doped monoliths. We have shown that the so-formed nanoparticles were able to resist to several heat-treatments of the glass at high temperature (about 2000°C) and that they could survive until the final fibre geometriy is obtained. The linear and non-linear optical properties of such fibres fabricated using these monoliths are clearly modified by the presence of nanoparticles.
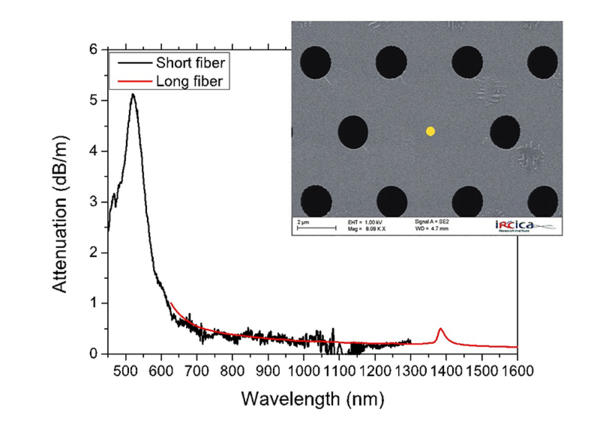
Attenuation curve of air/silica fibre with a core partially doped by gold NPs. Inset : SEM image of the fibre on wich the doped zonehas been symbolised in yellow.
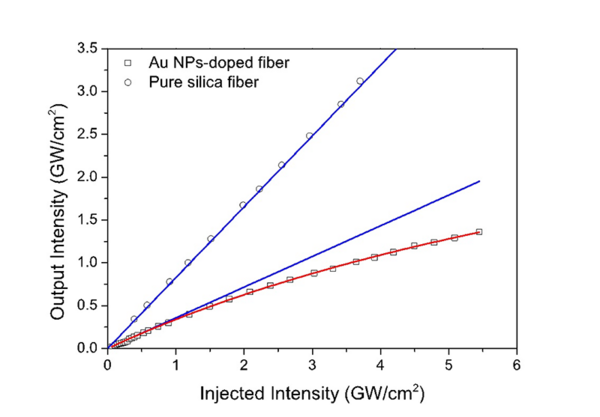
Curves illustrating the transmission response as a function of the input intensity in a gold NPs-doped or in an undoped air/silica fibre.
Another option consists in doping the porous gel or the glass with NPs precursors, then to prompt their crystal growth in a localised manner under a laser beam. In this domain, our team has acquired international acknowledgement. In particular in porous sol-gel silica, 2D or 3D gratings of metal nanocristals (Au, Ag) or semiconductors nanocrystals (CdS, PbS) have been generated in different irradiation conditions(pulsed or CW lasers at various wavelengths). Photo-thermal or photo-chemical mechanisms have been identified depending on the employed laser and precursors. This work, falling within the framework of a project supported by ANR POMESCO, has led us to define the best experimental conditions allowing efficient synthesis of stable NPs in silica xerogels. Extrapolation of these techniques to dense glasses, then to optical fibres, is going on, the localised aspect of the NPs growth being particuliarly interesting for the use of optical nonlinearities in pulsed lasers.
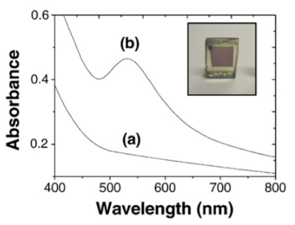
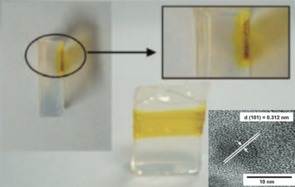
Left: Photograph and absorption spectra showing SPR resonance of gold NPs produced in silica xerogel under femtoseconde laser irradiation.
Right: Photograph and HRTEM image of CdS NPs produced in the vicinity of a gel surface by visible CW laser irradiation.
c) Fibres for dosimetry
The fields of health nuclear industry are nowadays enquirer of fibred technologies. In medicine for example, Beside the biomedical laser or fibrescopy, which are among the most widespread applications of optical fibre, these fibred technologies can lead to the achievement of reliable, precise and innovative measurement systems in order to offer to practitioners real-time useful tools, more and more efficient during medical interventions on patients. In the nuclear domain, dose or temperature metrology increasingly makes use remote sensors, among which optical fibre has a role to play in accordance with its immunity regarding parasitic electromagnetic waves. Silica fibres are particularly interesting because they are able to resist to harsh environments, for example to radiation doses of several kilograys (kGy). In this context, our team considers developing new doped silica rods, as well as new fibres for ionizing radiation metrology (X-ray, g, protons…). The operating modes of such devices are based on radio-induced luminescence phenomena, either spontaneous (Radioluminescence RL or scintillation) or optically stimulated (OSL). To generate this luminescence, pure silica preforms prepared by sol-gel process or by MCVD, then the corresponding fibres, have been doped with one or two doping species (Cu+, Ce3+, Tb3+, Gd3+ ions, germanium oxide …).
Fibred dosimeters should play a major role in the future in the area of radiotherapy and of high energy physics, being given their intrinsic advantages (radiation resistance, multi-core fibres, low intrusiveness, etc.). As an example, the conception of fibred dosimeters could allow localised and remote measurements of radiations, which would represent a real progress in cancer treatment by radiotherapy, but also in the survey of nuclear plants or for radioactive waste storage.
We have already demonstrated the potentiality of doped fibres for UV, X, g dosimetry and also under proton beam. The SURFIN project (new materials for the survey of nuclear plants through optical fibres), funded by ANDRA and French PIA over the period 2017-2022, is coordinated by PhLAM, performed in collaboration with Laboratoire Hubert Curien (LabHC) at University of Saint-Etienne, the Institut de Chimie de Clermont-Ferrand (ICCF) at University of Clermont-Auvergne and the Institut de Physique de Nice (InPhyNi) at University of Sophia Antipolis. Another project, this one having medical purpose, aims at exploiting the small dimensions of such optical fibres, as well as developing their sensitivity and their response dynamics, for a real-time and precise definition of dose or dose-rate profiles in radiotherapy X or in pulsed protontherapy. This is the reason of FIDELIO project (Fiber-based In-vivo Realtime Dosimetry for Pulsed Radiotherapy), funded by French ANR over the period 2021-2025 and to which we participate with InPhyNi, LabHC and the iXBlue Photonics Division firm in Lannion. Other research operations concerning proton beam dosimetry through optics fibres have been conducted in collaboration with the Canadian particle accelerator centre TRIUMF in Vancouver.
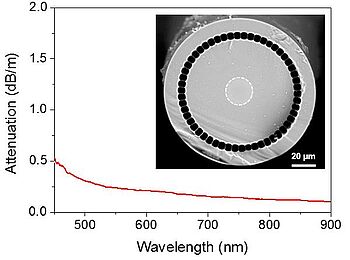
Attenuation spectrum of an air-clad structured fibre doped with cerium.
Radioluminescence of the fibre under X irradiation with a 1.2 Gy/s dose rate. RL response linearity as a function of the dose rate.